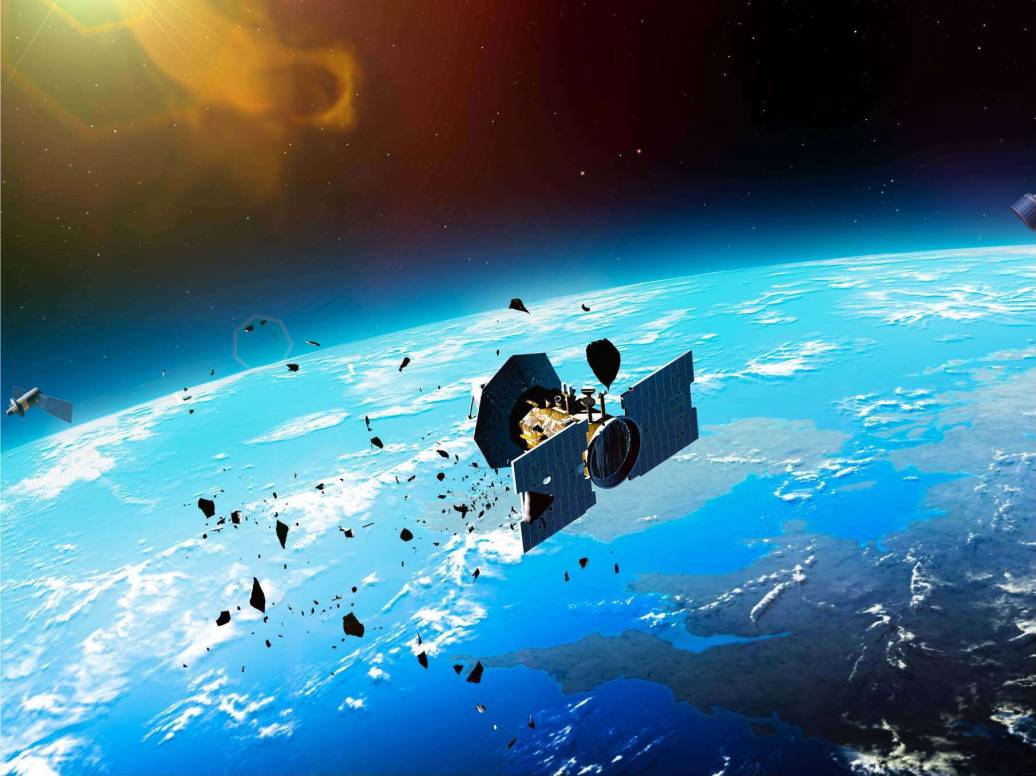
We need to talk about the space debris problem
A current lack of regulation, cooperation, automation and increased defence anxiety has made today’s systems unfit for future LEO activity
By Chris McKenzie, Futureworx Architect
Over the past 65 years, mankind has launched over 13,000 objects into Earth’s orbit, with approximately 8,000 satellites still in orbit, out of which 5,600 are currently active according to the latest figures provided by the European Space Agency’s (ESA) Space Debris Office. But the bigger picture is that this only represents a modest portion of the total number of objects orbiting our planet, the rest being debris of our legacy in space. This includes:
- Spent rocket stages
- Inactive satellites
- Stray bolts
- Paint chips
- Solid-rocket-motor slag and the scattered fragments from explosions, collisions and anti-satellite tests.
In total, there are approximately 30,000 large objects (greater than 10cm) and millions of smaller objects; the vast majority of these objects occupy the Low Earth Orbit (LEO), typically classified as a height of 200km to 2,000km above the Earth’s surface.
The current NASA view is that the best solution to managing debris in the long term is to strive for a 90% debris mitigation compliance and to actively remove five inactive spacecraft per year that would not naturally de-orbit.
The risk of Kessler Syndrome
The problem of space debris has been debated since the early years of space activity. In 1978, NASA Scientist Donald J. Kessler predicted a future scenario in which the density of objects in LEO would be high enough that collisions between objects could cause a cascade in which each collision generates space debris that increased the likelihood of yet further collisions, eventually making LEO an inaccessible belt of debris. The scenario became known as Kessler Syndrome.
While this future vision still seems a distant prospect, it has already been modelled by both NASA and ESA that we have passed a critical mass of debris where the amount of debris in LEO has grown to the point that even if no additional objects were launched into orbit, collisions would continue to occur, increasing debris at a higher rate than it naturally de-orbits in the thin atmosphere at lower altitudes (although it is worth noting this growth would occur gradually over decades).
These collisions are known as ‘fragmentation’ events, which can dramatically increase the number of objects in orbit. However, collisions are not the only fragmentation mechanism; in fact, the most common fragmentation events are explosions from left-over energy in fuel and batteries onboard spacecraft and rockets.
More recently, anti-satellite missile testing has resulted in significant step changes in the number of objects in orbit. The NASA chart below illustrates the increase in objects following collisions and anti-satellite tests. It’s worth noting this data does not include more recent anti-satellite tests by India in 2019 and Russia in 2021, which together added another 2,000 trackable objects.
Efforts have been underway for several years to address the problem of space debris, mostly concentrated on debris mitigation, i.e., limiting the generation of new debris. The Orbital Debris Mitigation Standard Practices (ODMSP) are voluntary guidelines developed by NASA in 2001 and later adopted by an international forum of space faring nations, the Inter-Agency Space Debris Coordination Committee (IADC), and then by the United Nations Committee on the Peaceful Uses of Outer Space (UNCOPUOS).
The broad objectives are:
- Control of debris released during normal operations
- Minimising debris generated by accidental explosions
- Selection of safe flight profile and operational configuration to minimise accidental collisions
- Post-mission disposal of space structures
The post-mission disposal objective targets a 25-year rule for atmospheric drag re-entry (which will naturally occur at altitudes below 600km), repositioned into storage/graveyard orbit or active removal within five years.
Compliance with these guidelines is very high in the geosynchronous orbit of Earth (GEO) due to the narrow altitude band creating a self-interest in debris mitigation; however, in LEO overall compliance is low. This is despite NASA achieving compliance above 95% and launch vehicles dramatically reducing their contribution (now approaching 90% compliance), thanks in part to the emergence of reusable vehicles.
The challenge of debris removal
While debris mitigation is our most effective strategy for managing debris in orbit, we have already reached a point where the debris population will continue to grow even with a cessation of space launches. Therefore, debris remediation, in the form of active debris removal, has started to be trialled.
There is no one-size-fits-all solution to this problem and a number of removal concepts have been investigated. Currently, Japanese orbital debris removal company Astroscale is demonstrating the magnetic grapple plate concept known as End-of-Life Services by Astroscale-demonstration (ELSA-d) and are working with global satellite communications network OneWeb on a commercial multi-client version called ELSA-m (End–of–Life Services by Astroscale-Multi).
The ESA is also working with Swiss start-up ClearSpace on spacecraft with four robotic arms to retrieve an upper stage of a 2013 launch vehicle. While technical solutions are now becoming a reality, active debris removal remains technically, legally, and commercially difficult.
The current NASA view is that the best solution to managing debris in the long term is to strive for a 90% debris mitigation compliance and to actively remove five inactive spacecraft per year that would not naturally de-orbit.
This large debris field is a daily headache for satellite operators, as well as the International Space Station (ISS) which orbits at an altitude of approximately 400km. ‘Conjunctions’ (that is, collisions) between objects in LEO have an average impact velocity of 17,500mph; therefore, spacecraft conjunctions with objects greater than 10cm would be catastrophic.
Indeed, objects greater than 1cm would be mission ending (damaging mission critical systems), with small objects still causing significant damage, with sufficient energy to crack windows and penetrate space suits. Satellites incorporate some level of protection of critical systems against very small debris – the ISS hull and critical systems can withstand debris up to approximately 1cm – but operators in Earth’s orbit remain exposed to the risk of mission ending conjunctions.
Managing orbital surveillance
To mitigate these conjunctions, objects in Earth’s orbit are tracked and catalogued using ground-based radar and optical telescopes as well as some satellites. The primary provider of this service is the US Department of Defense Space Surveillance Network, which currently track objects greater than 10cm. Other agencies, nations and commercial entrants are also increasing their capability.
In the event of a predicted conjunction, the affected operator will receive a ‘Conjunction Data Message’ (CDM). Due to the uncertainties in these tracking predictions, the risk level will be monitored for several weeks. If the risk continues to increase, an avoidance manoeuvre may be required (ESA’s threshold is a probability of greater than 1 in 10,000). For a typical satellite, hundreds of alerts can be received each week, with typically only a couple needing detailed follow-up analysis, resulting in at least one avoidance manoeuvre per year per satellite.
These manoeuvres use valuable fuel and typically take mission systems down for a period of time. For example, in 2021 an ESA Galileo constellation satellite performed a manoeuvre to avoid an inert Ariane 4 upper stage and was out of service for three weeks.
Regardless, there are significant problems with today’s system as the process is largely manual, ad-hoc, and un-coordinated. Additionally, only objects above 10cm are tracked, leaving spacecraft operators exposed to hundreds of thousands of smaller ‘Lethal Non-Trackable’ (LNT) objects.
The CDMs are also simplified, partially redacted (up to 25% are not shared due to military sensitivities) and not always timely. Simply put, the current system is not suitable for future projected demand.
Mega-constellations in ‘New Space’
But there’s an even bigger problem – ‘New Space’. Until recently, space activity had largely been limited to government space agencies due to the expense in technology development and launch costs. However, due largely to the efforts of SpaceX and their reusable launch vehicle Falcon 9, operational costs have come down considerably. This in turn has led to a significant increase in commercial entrants to LEO operations.
However, the past few years have seen a step change in new LEO objects with the arrival of ‘mega-constellations’ (as illustrated below). These are systems utilising a large network of LEO satellites that have the ability to maintain a global coverage despite the individual limited coverage of each satellite in their LEO orbit. This boom has been fuelled by the forecasted massive future demand for highspeed mobile data access.
SpaceX has again become the largest operator in the field, with currently 1,900 StarLink satellites in orbit, with a US license to launch 12,000 and the ambition to launch a total 42,000.
But they are not alone. OneWeb already has a smaller operational constellation; Amazon has plans for a large constellation called ‘Project Kuiper’; a Chinese state-owned consortium is planning a ‘GW’ constellation similar in size to StarLink; even established operators such as Canada’s Telesat plan to add constellations.
There are additional mega-constellation concerns with the high volume of satellites slowly de-orbiting at end-of-life adding to complexities of traffic management, as well as the large-scale release of alumina into the upper atmosphere upon re-entry. Astronomers also have concerns on the impact of their view of the night sky.
This disruptive explosion of objects in LEO sits in the context of practically no international regulation governing the management of this domain and to hold spacefaring entities accountable for conducting safe, responsible, and sustainable space operations. Instead, individual governments issue licences at their own discretion. This is in contrast to the tightly regulated GEO, overseen by the UN-backed ITU, which issues a finite number of slots to countries, who then divide them up for military, science, and commercial use.
This has been driven by the very narrow GEO orbital plane on which satellites are synchronous with Earth. In LEO, there are a range of viable altitudes, however the projected increased density of some orbit planes means the current approach may not be sustainable. There are also concerns that we may be witnessing a LEO 'land grab’ for orbital positions and radio frequencies.
The perils of future space use
In response to current surveillance deficiencies, projected increases in LEO objects and increased concerns around malicious defence activity in space, developments are underway to meet the needs of future space use.
The current space surveillance networks are largely a relic of the cold war ballistic missile warning systems, which are not optimised for tracking thousands of relatively small objects in orbit. Therefore, new dedicated sensors are being developed and deployed. The most notable is the US Space Fence system, developed by Lockheed Martin. This advanced ground-based phased-array radar can track objects down to 2cm and is now partially operational.
There are also significant commercial entrants, such as LeoLabs working towards similar tracking resolution capability, but free of the sensitivities of military ownership. However, these increased surveillance capabilities come with a significant increase in the number of objects to track and catalogue.
In view of the current deficiencies with the surveillance and the projected increase in objects to track, development programmes are underway to increase automation of processes. This includes tracking, conjunction analysis and risk assessment. Due to the large datasets involved, these systems are looking to Artificial Intelligence (AI) and Machine Learning technologies to help facilitate this capability.
The management of the StarLink constellation already relies on an AI autonomous collision avoidance system to keep its fleet away from other spacecraft based on CDM alerts. However, this has already introduced problems for other operators who have accused SpaceX of not being co-operative and open with the operation of its system.
Problems can also be created when automatic orbital adjustments change the forecasted trajectory and make conjunction predictions more complicated. The question, therefore, is this: could there be an argument for greater autonomy for individual satellites detecting and resolving conjunctions themselves, as well as co-ordinating traffic repositioning?
Whatever the solutions are, it’s clear we are at the beginning of a disruptive change in the use of LEO, and with a current lack of regulation, cooperation, automation and increased defence anxiety, there is much work to be done in making today’s systems and operations fit for future LEO activity.